Abstrakt: Tento článok skúma historický vývoj a súčasnú zložitosť riadenia letovej prevádzky, objasňuje úlohu technológie, regulácie a strategického plánovania. Článok mapuje začiatok riadenia letovej prevádzky od vizuálnej navigácie až po zavedenie radarových systémov a zdôrazňuje komplexný dizajn systému riadenia letovej prevádzky a výzvy, ktoré predstavujú exogénne a endogénne faktory. V článku sa nachádza podrobná analýza, pomocou metódy SWOT, kľúčových radarových technológií, vrátane primárneho radaru a sekundárneho radaru, spolu s novými technológiami, ako je multilaterácia širokej oblasti a automatické závislé sledovanie-vysielanie. Článok sa zameriava na budúce očakávania pre riadenie letovej prevádzky, berúc do úvahy rastúcu zložitosť spôsobenú rastom letectva. Príspevok sa zameriava najmä na prijatie ADS-B, analyzuje jeho transformačný potenciál, regulačné mandáty a obavy súvisiace s kybernetickou bezpečnosťou. Práca končí uznaním imperatívnej úlohy umelej inteligencie v budúcnosti riadenia letovej prevádzky. Napriek pokroku v informatike zostáva integrácia umelej inteligencie do manažmentu letovej prevádzky obmedzená, čo si vyžaduje ďalšie skúmanie pri zlepšovaní rozhodovacích procesov.
Kľúčové slová: riadenie letovej prevádzky, stratégia, kybernetická bezpečnosť, umelá inteligencia
JEL: L93
AIR TRAFFIC MANAGEMENT 2030 STRATEGY
Abstract: This paper explores the historical evolution and current complexities of Air Traffic Management, shedding light on the role of technology, regulation, and strategic planning. Tracing the beginning of Air Traffic Management from visual navigation to the introduction of radar systems, the paper emphasizes the complex design of the Air Traffic Management system and the challenges posed by exogenous and endogenous factors. A detailed analysis using the SWOT method of key radar technologies, including Primary Surveillance Radar and Secondary Surveillance Radar, along with emerging technologies like Wide Area Multilateration and Automatic Dependent Surveillance-Broadcast, is provided. The paper delves into the future expectations for Air Traffic Management, considering the increasing complexity driven by the growth of aviation. The paper particularly focuses on the adoption of ADS-B, analyzing its transformative potential, regulatory mandates, and concerns related to cybersecurity. The paper concludes by recognizing the imperative role of Artificial Intelligence in the future of Air Traffic Management. Despite advancements in computer science, the integration of Artificial Intelligence into Air Traffic Management remains limited, prompting the need for further exploration in enhancing decision-making processes.
Keywords: air traffic management, strategy, cybersecurity, artificial intelligence
1 Introduction
Even though the inaugural scheduled passenger flight in 1914 marked a pivotal moment in aviation history, Air Traffic Control (ATC) didn’t come into existence until later years. Pre-ATC, pilots relied on visual navigation techniques such as compasses and maps for flying and landing aircraft. The first ATC tower was introduced in 1920 at London’s Croydon Airport. Referred to as the “Aerodrome Control Tower”, its primary function was to provide basic traffic and weather guidance to pilots via radio [1]. The bedrock of this system is the Radar system. Designing and managing the Air Traffic Management (ATM) system involves a complex interplay of various factors. The European ATM system is intricately designed but is still significantly distant from an optimal state [2]. A fundamental aspect of its functionality centers on the coordination, collaboration and cross-border cooperation among Air Navigation Service Providers (ANSPs), which, in turn, are influenced by a combination of exogenous and endogenous factors. Exogenous factors involve elements beyond ANSPs’ direct control, including legal frameworks, socioeconomic conditions, operational constraints, and international regulatory obligations like those established by the Single European Sky initiative. In contrast, endogenous factors lie within ANSPs’ control, spanning organizational, managerial, financial, and operational aspects. This intricate mix of factors makes the strategic planning and development of such a system notably challenging [3]. For the management in question, two types of radars stand out: the Primary Surveillance Radar (PSR) and the Secondary Surveillance Radar (SSR) [4]. Other technologies, besides the PSR and SSR, such as the Wide Area Multilateration (WAM) and the Automatic Dependent Surveillance-Broadcast (ADS-B) are also used [5].
The Primary Surveillance Radar uses reflected radio signals. As its antenna rotates at an approximate velocity of 5-12 rpm it emits a pulse of radio wave. When this wave encounters an aircraft or another object, it gets reflected, and a portion of the energy is directed back to the antenna [6].
By using the polar coordinate system, it is able to obtain the range (by calculating the time difference between the emitted and received pulse) and bearing (via the antenna azimuth) of the targets found in respect of the antenna position. It has some limitations though:
- An area of airspace directly above the antenna, called cone of silence, remains unsurveyed due to the radar’s radiation pattern,
- Targets with the same slant range but at different levels are hard to distinguish, resulting in overlapping blips on the display,
- The radar cannot discern whether the reflected signals originate from aircraft or other objects like terrain, buildings, or clouds – such reflections are termed as clutter,
- Unambiguous range limit – the radar faces difficulty determining if a received signal corresponds to the most recent emitted pulse. This may lead to false targets [6].
The Secondary Surveillance Radar antenna rotates at an approximate velocity of 5-12 rpm and transmits a pulse that’s received by the transponder on board. It replies by sending a message containing at least a code (if operating in Mode A). However, commonly, this is supplemented with level (mode C) additional details such as aircraft identification, chosen altitude, etc. (Mode S). The data received depends on the interrogation mode (A, C or S) and capability of the transponder. Typically, two Mode A interrogations are followed by a Mode C interrogation. As the identity of the aircraft is of highest importance to the controller, the mode A is the one that is more frequently used [7].
Offering several benefits such as requiring much less power to attain the desired range comparing to PSR, with targets being easier to distinguish due to the different SSR codes and immunity to clutter due to the utilization of different frequencies for interrogation (1030 MHz) and replies (1090 MHz), SSR also has its drawbacks:
- It relies on the onboard equipment and in event of a transponder failure, de SSR will receive no reply, resulting in a lack of target knowledge,
- A phenomenon called “garbling” can take place when two replies are received at the same time (given that the aircraft’s slant range and bearings are identical) [7].
Combining the advantages of PSR and SSR is commonly practiced by co-locating them and using both [7]. Figure 1 shows the PSR and the SSR principles of operation.
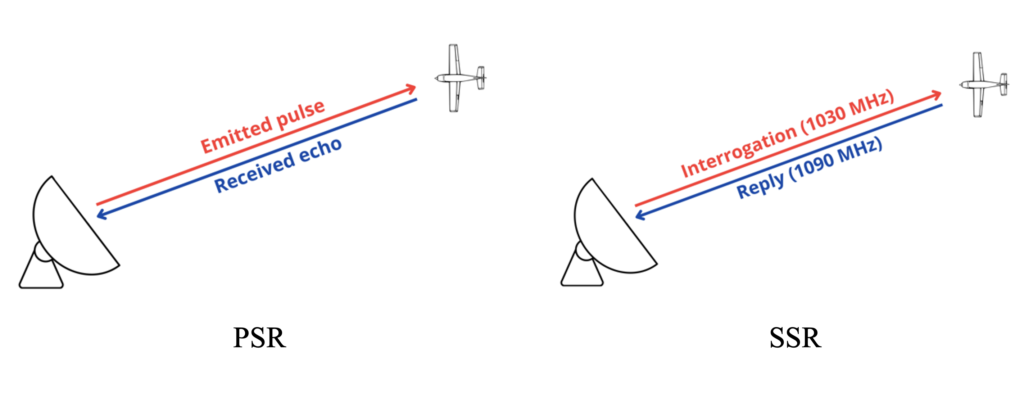
Source: Authors
Fig. 1. PSR and SSR principles of operation.
The Wide Area Multilateration utilizes a network of sensors spread across a designated area to monitor airspace, exploiting the 1090 MHz transmissions broadcast from aircraft [8]. It can be deployed in challenging terrain unsuitable for conventional radars. It can also function as a replacement for secondary radar or a supplementary tool in conjunction with ADS-B as addressed further in this paper [8]. WAM sensors collect data from aircraft avionics through either ADS-B squitters 1 or transponder responses. Additionally, WAM ground sensors have the capability to actively query transponders to generate responses, which are subsequently gathered and analyzed by the WAM system.
Automatic Dependent Surveillance – Broadcast stands out as a significant implementation system of the NextGen initiative, designed to enhance safety and efficiency in the National Airspace System by facilitating communication between pilots and air traffic controllers [9]. This advanced surveillance technology integrates an aircraft’s positioning source, avionics, and ground infrastructure to establish a precise surveillance interface between the aircraft and ATC [9]. Being different and more precise than radar it consists of two different services: ADS–B Out and ADS–B In.
ADS-B Out involves the periodic broadcast of information, including the aircraft’s GPS location, altitude, ground speed, and other relevant data, to ground stations and fellow aircraft at a rate of once per second. ADS-B In, however, delivers valuable weather and traffic position information directly to the cockpit for the operators [9].
2 Methodology
The paper aims to identify, at the current time, the strategic position and possibilities for the ATM up until the year 2030. Afterall, the strategy for Air Traffic Management until 2030 drove the research and literature review, leading to the analysis of multiple airlines and companies to reach multiple conclusions on what their plans are.
To gather pertinent literature for this study, a systematic approach was adopted using reputable academic databases, including Google Scholar, ResearchGate, Scopus, and Web of Science. Firstly, it was required to filter a multitude of articles to determine their relevance to the researched theme, and secondly, to assess their reliability, with a preference for contributions from established entities such as major airline companies. The objective was to cast a wide net and include as many articles as possible, ensuring a comprehensive and reliable foundation for the study. The primary challenge encountered during this phase was to discern if the information on the article was relevant and reliable amidst the abundance of available information. Emphasis was placed on extracting data from sources associated with prominent airline companies as mentioned earlier. The subsequent task involved organization of the gathered information. Additionally, a crucial aspect of the data collection process was the comparative analysis of information obtained from the various consulted articles. This methodological approach aimed to uphold the rigor and credibility of the data collection process, laying the groundwork for a analysis of the researched theme. The thoroughness of this procedure contributes to the reliability and validity of the study, ensuring that the data used for analysis is both relevant and from reputable sources within the aviation industry.
3 Results
The core of socio-economic structure revolves around mobility and its fundamental transport components, being them either air, inland, or maritime domains. In the contemporary context, the effectiveness, speed, interconnectivity, and inclusivity of mobility are predominant considerations to have into account [10].
According to United Nations projections, it is anticipated that, by 2050, two-thirds of the global population will be residing in cities. But one pertinent question arises: How is it possible to modify and elevate current, already-stretched mobility system to meet the evolving expectations and increased demands of the future? The field of aviation is abundant with innovations, from unmanned aircraft advancements, artificial intelligence, biometrics, robotics, blockchain to the development of alternative fuels and electric aircraft. This position is ideal for aviation to contribute to the ongoing discourse on innovation and its potential implications for new modes of transportation. The magnitude of air traffic may come as a surprise to some but, globally, aircrafts are taking off at a rate exceeding 400 departures per hour, and this statistic contains solely scheduled commercial traffic. Air transport serves as a conduit for the global movement of people and cargo [10]. The upcoming decades are anticipated to bring heightened complexity to ATM due to the huge growth and increased complexity of aviation. In order to hold up to the aviation safety standards, there is a pressing need for enhancements in the ATM domain [11]. Within the airspace of the United Arab Emirates, the total number of flights is expected to increase from 608 877 in 2010 to an estimated 1 533 409 by 2030. The average growth rates are forecasted to be 6.8% from 2010 to 2015, 4.8% from 2015 to 2020, and 3.7% from 2020 to 2030 [12]. It is, therefore, acknowledged that without substantial improvements in the ATM domain, the safety objectives that were outlined by international organizations are unlikely to be met, leading to a higher risk of incidents and accidents [11].
The adoption of automation and digital technologies is on the rise in air traffic control and management. With the usage of high-definition cameras, ADS-B technology, and remote sensing, the groundwork is being laid for the implementation of unmanned or autonomous digital towers. Through these advancements, remote centralized air control centers can receive images from camera and be able to present controllers with a comprehensive view of the airfield to enhance their situational awareness. Digital tower technologies contribute to enhance operational efficiency, safety, and flexibility. The centralization of operations not only boosts productivity but also reduces the maintenance requirements of systems and equipment [13].
ADS-B stands as a primary element within the next-generation of air transportation system. Mandatory for flight within the majority of controlled airspaces, equipping aircraft with ADS-B is imperative [13]. In contrast to conventional radar surveillance technologies, PSR and SSR, which rely on ground-based antennas to measure an aircraft’s range and bearing, ADS-B enables aircraft to determine their own position using a Global Navigation Satellite System. After such doing, this information is periodically broadcasted over a radio frequency to ground stations or nearby aircraft. A key advantage of NextGen lies in the continuous broadcast of critical flight information, including altitude, heading, and velocity. This diminishes the reliance on expensive and comparatively imprecise PSR and SSR systems, thereby significantly enhancing situational awareness for both pilots and air traffic controllers while simultaneously reducing air traffic surveillance costs [14].
In 2010, recognizing the transformative potential of ADS-B, the Federal Aviation Administration established a final rule mandating that all aircraft must be equipped with ADS-B by 2020. Additionally, the nonmechanical nature of ADS-B ground infrastructure allows for flexible siting in locations challenging for traditional radar installations. While WAM demands more ground stations and has larger geometric requirements compared to ADS-B, it gains an early implementation advantage by leveraging existing aircraft equipage [10]. While ADS-B enhances the capabilities of conventional air traffic surveillance systems, there exist several aspects of this technology that necessitate further elucidation and scrutiny to ensure its safe adoption. Notably, ADS-B has garnered significant media attention due to concerns about its security [15]. Seeking more recent information, it was found in a report by Aviation International News magazine of 2018 that the FAA had addressed numerous issues related to the 2020 ADS-B mandate, including pricing, technology availability, and paperwork requirements. However, comprehensive attention to cybersecurity issues associated with ADS-B technology was yet to be fully undertaken. To address concerns related to blocking, a proposed solution involved altering the aircraft ID transmitted by ADS-B, ensuring it no longer corresponds to the airplane’s registration information. This approach aimed to maintain the advantages of ADS-B while alleviating some of the apprehensions regarding aircraft identification [15].
The arrangement of ADS-B gives rise to supplementary concerns regarding data security. Within this configuration, ADS-B receivers installed in each aircraft collect location information from onboard GPS sensors, which, in turn, receive signals from GPS satellites. Subsequently, this location data is transmitted alongside information from other avionics to ADS-B receivers situated on the ground or in the proximity of other aircraft. The ground stations then relay the received data to air traffic control. Aircraft equipped with ADS-B In receivers can further obtain this information for traffic detection and capitalize on the enhanced functionalities enabled by ADS-B In, such as accessing free weather information. Nevertheless, it is crucial to note that at no stage in this process is the data encrypted, implying that anyone with a receiver has the capability to observe the transmitted data. There is also an absence of authentication requirements. All these vulnerabilities expose the aircraft to potential cyber-attacks. The most well-known cyber-attack against ADS-B systems involves the act of spoofing where false data is inserted into communication pathways, creating illusions of non-existent aircraft or misleading locations [15]. Given the system’s exclusive reliance on GNSS data, lacking traditional radar, a concern arises due to the absence of means for receivers of ADS-B information to independently verify the accuracy of the transmitted data. Addressing this, it’s crucial to focus on validating ADS-B data against alternative technologies to rectify potential inaccuracies [14]. For example, in order to contradict the problems referred above, one of the solutions involved the establishment of a large-scale ADS-B Sensor Network for Research known as OpenSky. Historically, the extensive real-world data was limited to an exclusive group of industrial and governmental entities due to the necessity for specialized and costly equipment. Initiatives, for example, as OpenSky, utilizing “off-the-shelf” ADSB sensors distributed among volunteers across Central Europe, have democratized access. Researchers can now analyze billions of ADS-B messages, marking a significant shift in data accessibility for comprehensive analysis [14].
The innovative air-to-ground communication system relies on adaptive modulation and beamforming facilitated by the integration of automatic dependent surveillance—broadcast and multilateration techniques. The proposed multilateration technique for aircraft geolocation utilizes time-difference-of-arrival, angle-of-arrival, and frequency-difference-of-arrival features in the ADS-B signal. This hybrid approach selects multilateration sensors, being capable of minimizing geometric dilution-of-precision to increase accuracy and resilience against attacks like aircraft impersonation and ADS-B message infringement. A hardware prototype is expected to have an accuracy in the angle-of-arrival of 1.5° and a validated geolocation accuracy of approximately 30m over 100 nautical miles [16].
To enhance the performance of ATG systems, achieving highly accurate real-time geolocation estimation for aircraft is imperative. Traditional aircraft surveillance techniques involve the use of a primary surveillance radar and a secondary surveillance radar, which are supposed to be replaced by the ADS-B technology by the end of 2025 [17]. Being unauthenticated and unencrypted signal, providing open visibility to all aircraft within the airspace as mentioned before, ADS-B is susceptible to security vulnerabilities such as the lack of data authentication as protection against unauthorized message injection from and the absence of message encryption to counter spying and aircraft impersonation [17]. Various approaches have been proposed to address these challenges, such as estimating the angle-of-arrival of the ADS-B signal and among these approaches is the implementation of ADS-B systems in conjunction with wide-area multilateration, which relies on capturing the ADS-B signal across widely separated sensors for independent aircraft location estimation, decoupled from conventional ADS-B signal demodulation [18]. The integration of ADS-B transponders with the multilateration technique presents a more reliable and cost-effective option for meeting the requirements of future air traffic management systems. Anticipated to complement existing SSR infrastructure at airports, it is expected that WAM, combined with ADS-B, will play a supportive role in the evolving air traffic management. The utilization of TDOA assisted with WAM in conjunction with ADS-B offers a considerably more precise aircraft location estimate compared to traditional PSR or SSR. This approach is not only cost-effective but also enhances aircraft tracking and detection performance significantly in comparison to the SSR architecture [17]. This results in a substantial enhancement of aircraft tracking and detection performance in comparison to the SSR architecture, consequently advancing the overall capability of Air Traffic Management.
The impending complexity in ATM due to the expanding and intricate nature of aviation necessitates substantial enhancements to uphold aviation safety standards. It is acknowledged that without significant improvements in this domain, the safety objectives outlined by international organizations cannot be achieved, potentially leading to an elevated risk of incidents or accidents. Nowadays, computer science plays an essential role in data management and decision making within ATM. However, despite these advancements, the integration of Artificial Intelligence (AI), an extensively researched side of computer science, into the ATM domain remains relatively limited [11]. In the contemporary era, AI is the mantra, resonating among technologists, academics, journalists, and capitalists [19]. On a global scale, the domain of AI in ATM exhibits growth. The number of AI in ATM publications, has nearly doubled between the peaks of 2014 and 2018, experiencing over three times increase since 2010 across different categories [11].
The organizational framework of ATC systems is supposed to find significant evolution in the coming years, marked by the integration of AI and the proliferation of unmanned aerial vehicles. The development of new software customized for large-scale data analysis is underway, focusing on optimizing safe flight systems to mitigate risks and minimize delays [20]. Fortune Business Insight’s long-term projection for the global commercial drone market anticipates a substantial 25.07% compound annual growth rate from €1.33 billion in 2019 to €7.11 billion in 2027 [20].
Unmanned aerial vehicles and unmanned aerial vehicles traffic management are gathering attention for diverse applications such as package delivery, aerial mapping, agriculture and surveillance, particularly relying on line-of-sight. Unmanned aerial vehicles traffic management is indispensable for ensuring the safe operation of multiple fully autonomous unmanned aerial vehicles beyond the visual line of sight in a future environment characterized by dense traffic. Globally, various research and development teams are actively engaged in UTM initiatives, collaborating with different industrial partners for platform testing. The envisioned future scenario involves urban airspace congested with various types of autonomous aerial vehicles, posing challenges to ATM, which are expected to be addressed through AI-assisted solutions [21].
4 Conclusion
By exploring the evolving landscape of Air Traffic Management and addressing the challenges and opportunities presented by the advancements in technology, it is possible to realize the pressing need for substantial enhancements in ATM to ensure aviation safety standards are met in the face of the expanding and intricate nature of aviation. The integration of technologies such as PSR, SSR, WAM and ADS-B is crucial for efficient surveillance and tracking. Also, the rise of AI in every field, including ATM, is evident, with a growing number of publications reflecting increased attention to this field. Strategic initiatives anticipate regulatory changes and improve safety. The challenges associated with cybersecurity and data validation in technologies like ADS-B are acknowledged by the world and the need is much for immediate and comprehensive solutions. As the paper delves more into the future, there’s recognition on the importance of technology coupling, such as ADS-B with WAM and the role of AI in shaping a safer and more efficient airspace. Overall, a comprehensive overview of the technological, operational and strategic facets within the realm of ATM, laying the foundation for an adaptive and resilient future in air traffic.
In conclusion, this exploration of ATM complexities and technological advancements underscores the need for continued innovation, collaboration, and strategic planning within the aviation industry. Embracing emerging technologies while addressing associated challenges is pivotal for fostering a robust, safe, and efficient air traffic management system capable of meeting the demands of the future.
5 References
- FRIEDRICH, M. – BIERMANN, M. – GONTAR, P. – BIELLA, M. – BENGLER, K. The influence of task load on situation awareness and control strategy in the ATC tower environment. In Cognition, Technology & Work. 2018. Vol. 20, p. 205-217. DOI: 10.1007/s10111-018-0464-4.
- BUYLE, S. – DEWULF, W. – KUPFER, F. – ONGHENA, E. – MEERSMAN, H. – VAN DE VOORDE, E. Van de Voorde, E. From traditional to professional Air Navigation Service Provider: A typology of European ANSP business models. In Journal of Air Transport Management. 2021. Vol. 91, p. 102006. DOI: 10.1016/j.jairtraman.2020.102006.
- REZO, Z. – STEINER, S. – MIHETEC, T. – ČOKORILO, O. Strategic planning and development of Air Traffic Management system in Europe: A capacity-based review. In Transportation Research Procedia. 2023. Vol. 69, p. 5–12. DOI: 10.1016/j.trpro.2023.02.138.
- CONSTANTINESCU, N. – CONSTANTINESCU, E. – CHIRA, A. I. Enhancing the performance of the Primary Surveillance Radar using Multilateration. In Incas Bulletin. 2022. Vol. 14, p. 35– 49. DOI: 10.13111/2066-8201.2022.14.4.4.
- POURVOYEUR, K. – MATHIAS, A. – HEIDGER, R. Investigation of measurement characteristics of MLAT / WAM and ADS-B. In Tyrrhenian International Workshop on Digital Communications – Enhanced Surveillance of Aircraft and Vehicles. 2011. pp. 203-206.
- STAHL, H. – FISCHER, H. – STANZEL, S. – KLÖCK, C. – BERNHARDT, F. – KOUEMOU, G. Evaluation of passive multi-static primary surveillance radar for operational use in civil air traffic control. In 2018 19th International Radar Symposium. 2018. pp. 1-10. DOI: 10.23919/IRS.2018.8448044.
- CAMPUS, M. I. T. – CHROMEPET, C. Air Traffic Control Secondary Radar. In International Journal of Pure and Applied Mathematics. 2018. Vol. 119, p. 2935-2944.
- KAUNE, R. – STEFFES, C. – RAU, S. – KONLE, W. – PAGEL, J. Wide area multilateration using ADS-B transponder signals. In 2012 15th International Conference on Information Fusion. 2012. pp. 727-734.
- OLAGANATHAN, R. Safety Analysis of Automatic Dependent Surveillance–Broadcast (ADS-B) System. In International Journal of Aerospace and Mechanical Engineering. 2018. Vol. 5, p. 9-18.
- ABEYRATNE, R. No Country Left Behind and ICAO’s Leadership Role in Air Transport. In Air and Space Law. 2022. Vol. 47, p. 315-330. DOI: 10.54648/aila2022017.
- DEGAS, A. – ISLAM, M. R. – HURTER, C. – BARUA, S. – RAHMAN, H. – POUDEL, M. – RUSCIO, D. – AHMED, M. U. – BEGUM, S. – RAHMAN, M. A. – BONELLI, S. – CARTOCCI, G. – DI FLUMERI, G. – BORGHINI, G. – BABILONI, F. – ARICÓ, P. A Survey on Artificial Intelligence (AI) and eXplainable AI in Air Traffic Management: Current Trends and Development with Future Research Trajectory. In Applied Sciences. 2022. Vol. 12, p. 1295. DOI: 10.3390/app12031295.
- SESHADRI, U. – KUMAR, P. – VIJ, A. – NDLOVU, T. Marketing strategies for the tourism industry in the United Arab Emirates after the COVID-19 era. In Worldwide Hospitality and Tourism Themes. 2023. Vol. 15, p. 169-177. DOI: 10.1108/WHATT-10-2022-0120.
- ROUSSET, E. Air traffic management and control systems for the aviation industry. In Airport Technology. 2021. Available at: https://www.airport-technology.com/buyers-guide/air-traffic-management-control-systems/.
- SCHAFER, M. – STROHMEIER, M. – LENDERS, V. – MARTINOVIC, I. – WILHELM, M. Bringing up OpenSky: A large-scale ADS-B sensor network for research. In Proceedings of the 13th International Symposium on Information Processing in Sensor Networks. 2014. p. 83–94. DOI: 10.1109/IPSN.2014.6846743.
- SEMENOV, S. – ZHANG, M. J. Comparative studies of methods for improving the cyber security of unmanned aerial vehicles with the built-in ADS-B system. In Advanced Information Systems. 2022. Vol. 6, p. 69-73. DOI: 10.20998/2522-9052.2022.4.10.
- NIJSURE, Y. A. – KADDOUM, G. – GAGNON, G. – GAGNON, F. – YUEN, C. – MAHAPATRA, R. Adaptive Air-to-Ground Secure Communication System Based on ADS-B and Wide-Area Multilateration. In IEEE Transactions on Vehicular Technology. 2016. Vol. 65, p. 3150–3165. DOI: 10.1109/TVT.2015.2438171.
- RUDYS, S. – ALEKSANDRAVICIUS, J. – ALEKSIEJUNAS, R. – KONOVALTSEV, A. – ZHU, C. – GREDA, L. Physical layer protection for ADS-B against spoofing and jamming. In International Journal of Critical Infrastructure Protection. 2022. Vol. 38, p. 100555. DOI: 10.1016/j.ijcip.2022.100555.
- JANA, S. – KASERA, S. K. On Fast and Accurate Detection of Unauthorized Wireless Access Points Using Clock Skews. In IEEE Transactions on Mobile Computing. 2010. Vol. 9, p. 449–462. DOI: 10.1109/TMC.2009.145.
- JORDAN, M. I. Artificial Intelligence—The Revolution Hasn’t Happened Yet. In Harvard Data Science Review. 2019. DOI: 10.1162/99608f92.
- HÖHROVÁ, P. – SOVIAR, J. – SROKA, W. Market Analysis of Drones for Civil Use. In LOGI – Scientific Journal on Transport and Logistics. 2023. Vol.14, p. 55–65. DOI: 10.2478/logi-2023- 0006.
- SHRESTHA, R. – BAJRACHARYA, R. – KIM, S. 6G Enabled Unmanned Aerial Vehicle Traffic Management: A Perspective. In IEEE Access. 2021. Vol. 9, p. 91119–91136. DOI: 10.1109/ACCESS.2021.3092039.
Acknowledgement
The paper is an output of the project KEGA 040ŽU – 4/2022 Transfer of progressive methods of education to the study program “Aircraft Maintenance Technology” and “Air Transport”.
Authors:
Kristína KOVÁČIKOVÁ 1, Andrej NOVÁK 2
Titles and place of work of the authors:
1Ing. Kristína Kováčiková, Air Transport Department, Faculty of Operation and Economics of Transport and Communications, University of Zilina, Univerzitna 8215/1, 010 26 Žilina, Slovakia, E-mail: kristina.kovacikova@stud.uniza.sk
2prof. Ing. Andrej Novák, PhD., Air Transport Department, Faculty of Operation and Economics of Transport and Communications, University of Zilina, Univerzitna 8215/1, 010 26 Žilina, Slovakia, E-mail: andrej.novak@uniza.sk